Biological dispersal
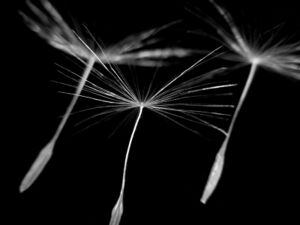
Biological dispersal refers to those processes by which a species maintains or expands the distribution of a population. Dispersal implies movement—movement away from an existing population (population expansion) or away from the parent organism (population maintenance). This is necessary for members of a population because organisms of the same age require all of the same resources within an ecosystem. Dispersal relieves pressure for resources in an ecosystem, and competition for these resources may be a selection factor for dispersal mechanisms.[1]
In the latter case, dispersal may simply involve replacement of the parent generation by the new generation, with only minor changes in geographic area occupied. More significantly, dispersal enables the species population to occupy much of the available habitat, thereby maximizing resources in its favor and providing a hedge against local adverse events.
In most cases, organisms (plants and especially sedentary animals) have evolved adaptations for dispersal that take advantage of various forms of kinetic energy occurring naturally in the environment: water flow, wind, falling (response to gravity).
Dispersal of organisms is a critical process for understanding both geographic isolation in evolution and the broad patterns of current geographic distributions.
Unlike animals, plants are limited in their ability to seek out favorable conditions for life and growth. Consequently, plants have evolved many ways to disperse and spread a population through their seeds or spores (see also vegetative reproduction). Those properties or attributes that promote the movement of the next generation away from the parent plant may involve the fruit more so than the seeds themselves.
Dispersal is a universal biological need, and it is to be expected that most higher plants have solved the problem in one way or another through adaptations involving their fruit or seed. Examine the fruit of any species and it is likely, with perhaps a bit more knowledge about the ecosystem, to at least intelligently speculate on what these adaptations are in that plant. However, realize that particularly where plant-animal interactions are central to the dispersal mechanism, seeing a plant outside of its native ecosystem may not reveal so much about the adaptations present in the fruit and seed. Indeed, in many instances of plants introduced into areas where they are not native, it is the failure of the dispersal mechanism that accounts for the species not becoming established beyond the garden.
Gravity (including wind)
The effect of gravity on the dispersal of seeds and spores is straightforward. Heavier seeds will tend to drop downward from the parent plant, and not very far by themselves. Spores, being much lighter, are more influenced by physical movements in the environment, especially those of wind ("anemochory") and water, and therefore less strictly subject to the simple motion of gravity (see examples below). Gravity may be sufficient agent for plants growing on steep slopes, but upslope movement of a population can be a problem. The naked seeds of gymnosperms are largely dependent upon gravity for dispersal. Most extant conifers are long-lived large shrubs or tall trees, thus taking full advantage of gravitational dispersal and allowing for gradual up slope movement of a population. Dispersal of seeds strictly by gravity should not overlook storm effects: seeds from a deteriorating cone growing high on a tall, narrow tree will get spread widely during a wind storm (see Wind below).
Encasing seeds in a rounded fruit promotes gravity driven movement away from the parent.
Mechanical
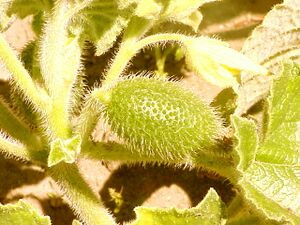
Numerous species have evolved mechanical means to overcome the tendency of a seed to drop close to its parent. Seedpods are often shaped so that the seeds are flung away from the parent plant with considerable force as the seedpod matures
Examples of fruit with mechanical dispersal mechanisms:
- Oxalis corniculata – capsule, as it dries, becomes sensitive to disturbance, ejecting tiny seeds in an explosive discharge.
Explosions
Plants that grow in water (aquatic and obligate wetland species) are likely to utilize water to disperse their seeds. For example, all mangroves disperse their offspring by water. Hydrochory is the dispersal of seeds by water; a plant which uses this method in its life cycle is termed a hydrochore. Rhizophora demonstrates an unorthodox method of propagation called vivipary: the embryo is retained on the plant until after germination; in essence, a dry seed is not produced. The hypocotyl of the germinating seedling (now called a propagule) bursts through the fruit and hangs, poised for continued growth. In R. mangle, the hypocotyl can reach a length of 20 to 25 cm; and in R. mucronata lengths up to 1 m have been recorded. Eventually, the seedling separates from the fruit, leaving its cotyledons behind, and—floating horizontally on the water surface—is carried away by tidal or river flow. After a month or two, the propagule turns vertical in the water. Once the hypocotyl of a propagule "feels" bottom or strands, roots start to develop and leaves appear at the upper end (Hogarth, 1999).
Adaptations commonly seen in littoral plants are those that promote flotation of the fruit, allowing the seed to be carried away on the tide or ocean currents. Examples would be:
- Cocos nucifera – the coconut produces a large, dry, fiber-filled fruit (a fibrous drupe) capable of a long survival adrift at sea.
- Calophyllum inophyllum – Alexandrian laurel or kamani produces a globose fruit that is almost cork-like.
Terrestrial plants may also have their seeds dispersed by raindrops.
By animals
A significant aspect of plant-animal co-evolution involves plant adaptations that take advantage of animal abilities to locomote. Some fruit have prickly burrs or spikes that attach themselves to a passing animal's fur or feathers so that the animal will carry them away. Some seeds are contained within a soft fruit that "invites" animals to consume it. These seeds have a tough protective outer-coating so that while the fruit is digested, the seeds will pass through their host's digestive tract intact, and grow wherever they fall. Such fruit attractive to birds is perhaps the most successful of fruit adaptations related to plant dispersal. Some seeds are appealing to rodents (such as squirrels) who hoard them in hidden caches, often beneath the surface of the soil, in order to avoid starving during the winter and early spring. Those seeds that are left uneaten have the chance to germinate and grow into a new plant.
Examples of fruits with attachment hairs and structures:
- Bidens spp. – Many species of this beggartick genus produce achenes with awns that are barbed, Medicago with rolled fruits bearing thorns and spikes, many Apiaceae with spikes or spiky hairs on their fruits like Torilis, Caucalis Daucus etc,
Some animals that disperse may also eat the seed.
Of animals
Most (but not all) animals are capable of locomotion and the basic mechanism of dispersal is movement from one place to another. Locomotion allows the organism to "test" new environments for their suitability, provided they are within animal's range. Movements are usually guided by inherited behaviors.
Non-motile animals
There are numerous animal forms that are non-motile, such as sponges, bryozoans, tunicates, sea anemones, corals, and oysters. In common, they are all either marine or aquatic. It may seem curious that plants have been so successful at stationary life on land, while animals have not, but the answer lies in the food supply. Plants produce their own food from sunlight and carbon dioxide—both generally more abundant on land than in water. Animals fixed in place must rely on the surrounding medium to bring food at least close enough to grab, and this occurs in the three-dimensional water environment, but with much less abundance in the atmosphere. However, that such a life form might be possible is at least suggested by the orb-weaver spiders.
All of the marine and aquatic invertebrates whose lives are spent fixed to the bottom (more or less; anemones are capable of getting up and moving to a new location if conditions warrant) produce dispersal units. These may be specialized "buds", or motile sexual reproduction products, or even a sort of alteration of generations as in certain cnidaria.
Corals provide a good example of how sedentary species achieve dispersion. Corals reproduce by releasing sperm and eggs directly into the water. These release events are coordinated by lunar phase in certain warm months, such that all corals of one or many species on a given reef will release on the same single or several consecutive nights. The released eggs are fertilized, and the resulting zygote develops quickly into a multicellular planula. This motile stage then attempts to find a suitable substratum for settlement. Most are unsuccessful and die or are fed upon by zooplankton and bottom dwelling predators such as anemones and other corals. However, untold millions are produced, and a few do succeed in locating spots of bare limestone, where they settle and transform by growth into a polyp. All things being favorable, the single polyp grows into a coral head by budding off new polyps to form a colony.
Motile animals
Although motile animals can, in theory, disperse themselves by their locomotive powers, a great many species utilize the existing kinetic energies in the environment. Dispersal by water currents is especially associated with the physically small inhabitants of marine waters known as zooplankton. The term plankton comes from the Greek, πλαγκτον, meaning "wanderer" or "drifter".
External links
Reference
- ↑ Irwini, AJ; PD Taylor (2000). "Evolution of Dispersal in a Stepping-Stone Population with Overlapping Generations". Theoretical Population Biology (Academic Press) 58: 321�328.
- Hogarth, Peter J. 1999. The Biology of Mangroves. Oxford Univ. Press. 228 p. ISBN 0-19-850222-2